A strategy to optimize soil phosphorus reserve: A study based on native maize of Mexico and genotype selection
DOI:
https://doi.org/10.17268/sci.agropecu.2024.026Keywords:
Zea mays L., cluster analysis, principal component analysis, germplasm, seedAbstract
Continual removal of phosphorus (P) by the crop gradually decreases soil fertility and drives the need for fertilizer inputs for agricultural production. Phytate is considered as the major storage pool for P in the seeds, but its contribution to human nutrition is low. The objective of this study was to characterize quantitative P concentrations (phytate, inorganic P and total P) of 50 native maize genotypes of Zea mays L. of the Patzcuaro lake, Michoacan, Mexico. The average total P and phytate concentration observed across all the genotypes was 3,1, 2,64, and 0,43 mg g-1, respectively. The relationship between phytate and total P was strong (r= 0,98**). This clearly suggests that with an increase in total P there is a corresponding increase in phytate concentration. Cluster analysis of data indicated five distinct groups with 1, 14, 11, 15 and 9 genotypes, respectively. Clusters I and II contained low phytate genotypes (1,74 mg g-1) while cluster V grouped most of the high phytate (4,9 mg g-1) contenting genotypes. Principal component analysis and F test showed the attributes more influencing group separation. Those attributes were total P and phytate. The first two components explained the 95% of the accumulation variation among groups. The race of the genotypes, however, was no clearly related to the group differentiation. These observations provide the first study on the genetic variety for breeding maize with lower P concentration to reduce P extraction from maize field at harvest.
References
Astier, M., Pérez-Agiz, E., Orozco-Ramírez, Q., Patricio-Chávez, M., & Moreno-Calles, A. I. (2012). Sistemas agrícolas, conocimiento tradicional y agrobiodiversidad: El maíz en la cuenca del Lago de Pátzcuaro. Conocimiento tradicional, innovación y reapropiación social, 146-172.
Bai, Z., Li, H., Yang, X., Zhou, B., Shi, X., et al. (2013). The critical soil P levels for crop yield, soil fertility and environmental safety in different soil types. Plant and Soil, 372, 27-37. https://doi.org/10.1007/s11104-013-1696-y
Bayuelo-Jiménez, J. S., & Ochoa-Cadavid, I. (2018). Interacción Genotipo x Ambiente para Eficiencia en el Uso de Fósforo en Maíz Nativo de la Meseta Purhépecha. Revista Fitotecnia Mexicana, 41(1), 39-47. https://doi.org/10.35196/rfm.2018.1.39-47
Bhagyawant, S. S., Bhadkaria, A., Gupta, N., & Srivastava, N. (2018). Impact of phytic acid on nutrient bioaccessibility and antioxidant properties of chickpea genotypes. Journal of Food Biochemistry, 42(6), 1-9. https://doi.org/10.1111/jfbc.12678
Bilal, H. M., Aziz, T., Maqsood, M. A., & Farooq, M. (2019). Grain phosphorus and phytate contents of wheat genotypes released during last 6 decades and categorization of selected genotypes for phosphorus use efficiency. Archives of Agronomy and Soil Science, 65(6), 727-740. https://doi.org/10.1080/03650340.2018.1521957
Bloot, A. P. M., Kalschne, D. L., Amaral, J. A. S., Baraldi, I. J., & Canan, C. (2023). A review of phytic acid sources, obtention, and applications. Food Reviews International, 39(1), 73-92.
Bünemann, E. K. (2015). Assessment of gross and net mineralization rates of soil organic phosphorus–A review. Soil Biology and Biochemistry, 89, 82-98.
Chí-Sánchez, F. A., Alvarado-López, C. J., Cristóbal-Alejo, J., González-Moreno, A., & Reyes-Ramírez, A. (2021). Contenido mineral de maíces criollos de Yucatán: análisis mediante μ-Fluorescencia de Rayos X. Terra Latinoamericana, 39, 1-12. https://doi.org/10.28940/terra.v39i0.454
Cong, W. F., Suriyagoda, L. D., & Lambers, H. (2020). Tightening the phosphorus cycle through phosphorus-efficient crop genotypes. Trends in Plant Science, 25(10), 967-975. https://doi.org/10.1016/j.tplants.2020.04.013
Dwivedi, S. L., Garcia-Oliveira, A. L., Govindaraj, M., & Ortiz, R. (2023). Biofortification to avoid malnutrition in humans in a changing climate: Enhancing micronutrient bioavailability in seed, tuber, and storage roots. Frontiers in Plant Science, 14, 1-24. https://doi.org/10.3389/fpls.2023.1119148
Frittelli, A., Botticella, E., Palombieri, S., Masci, S., Celletti, S., Fontanella, M. C., ... & Sestili, F. (2023). The suppression of TdMRP3 genes reduces the phytic acid and increases the nutrient accumulation in durum wheat grain. Frontiers in Plant Science, 14, 1079559.
Galván-Tejeda, N. D., Peña-Ramírez, V., Mora-Palomino, L., & Siebe, C. (2014). Soil P fractions in a volcanic soil chronosequence of Central Mexico and their relationship to foliar P in pine trees. Journal of Plant Nutrition and Soil Science, 177, 792–802.
González-Esquivel, C. E., Gavito, M. E., Astier, M., Cadena-Salgado, M., del-Val, E., Villamil-Echeverri, L., Merlín-Uribe, Y., & Balvanera, P. (2015). Ecosystem service trade-offs, perceived drivers, and sustainability in contrasting agroecosystems in central Mexico. Ecology and Society, 20(1), 38. http://dx.doi.org/10.5751/ES-06875-200138
Gunathunga, C., Senanayake, S., Jayasinghe, M. A., Brennan, C. S., Truong, T., Marapana, U., & Chandrapala, J. (2024). Germination Effects on Nutritional Quality: A Comprehensive Review of Selected Cereal and Pulse Changes. Journal of Food Composition and Analysis, 106024. https://doi.org/10.1016/j.jfca.2024.106024
Han, Y., White, P. J., & Cheng, L. (2022). Mechanisms for improving phosphorus utilization efficiency in plants. Annals of botany, 129(3), 247-258. https://doi.org/10.1093/aob/mcab145
HarvestPlus. (2022). Responding to crisis, building resilience: HarvestPlus 2021 annual report. HarvestPlus Annual Report 11. Washington, DC: International Food Policy Research Institute (IFPRI). https://ebrary.ifpri.org/cdm/ref/collection/p15738coll2/id/136342
Heuer, S., Gaxiola, R., Schilling, R., Herrera‐Estrella, L., López‐Arredondo, D., Wissuwa, M., ... & Rouached, H. (2017). Improving phosphorus use efficiency: a complex trait with emerging opportunities. The Plant Journal, 90(5), 868-885. https://doi.org/10.1111/tpj.13423
Houssni, I. E., Zahidi, A., Khedid, K., & Hassikou, R. (2024). Review of processes for improving the bioaccessibility of minerals by reducing the harmful effect of phytic acid in wheat. Food Chemistry Advances, 4, 100568. https://doi.org/10.1016/j.focha.2023.100568
Instituto Nacional de Estadística, Geografía e Informática (INEGI). (2017). Anuario estadístico y geográfico de Michoacán de Ocampo 2017. https://www.inegi.org.mx/contenidos/productos/prod_serv/contenidos/espanol/bvinegi/productos/nueva_estruc/anuarios_2017/702825092092.pdf
Jaradat, A., & Goldstein, W. (2018). Diversity of maize kernels from a breeding Program for Protein Quality III: Ionome Prof iling. Agronomy, 53, 956-976. https://doi.org/10.3390/agronomy8020009
Kafle, A., Cope, K. R., Raths, R., Krishna Yakha, J., Subramanian, S., Bücking, H., & Garcia, K. (2019). Harnessing soil microbes to improve plant phosphate efficiency in cropping systems. Agronomy, 9(3), 127. https://doi.org/10.3390/agronomy9030127
Kenzhebayeva, S., Abekova, A., Atabayeva, S., Yernazarova, G., Omirbekova, N., et al. (2019). Mutant lines of spring wheat with increased iron, zinc, and micronutrients in grains and enhanced bioavailability for human health. BioMed Research International, 2019, 1-11. https://doi.org/10.1155/2019/9692053
Mo, Q., Li, Z. A., Sayer, E. J., Lambers, H., Li, Y., et al. (2019). Foliar phosphorus fractions reveal how tropical plants maintain photosynthetic rates despite low soil phosphorus availability. Functional Ecology, 33(3), 503-513. https://doi.org/10.1111/1365-2435.13252
Murphy, J., & Riley, J. P. (1962). A modified single solution method for the determination of phosphate in nature waters. Analytical Chemistry Acta, 27, 31-36. https://doi.org/10.1016/S0003-2670(00)88444-5
Nadeem, M., Mollier, A., Morel, C., Vives, A., Prud’homme, L., & Pellerin, S. (2011). Relative contribution of seed phosphorus reserves and exogenous phosphorus uptake to maize (Zea mays L.) nutrition during early growth stages. Plant and Soil, 346, 231-244. https://doi.org/10.1007/s11104-011-0814-y
Nicholson, C. C., Emery, B. F., & Niles, M. T. (2021). Global relationships between crop diversity and nutritional stability. Nature communications, 12(1), 5310. https://doi.org/10.1038/s41467-021-25615-2
Ntswane, M., Labuschagne, M., Shandu, S. F., & Mbuma, N. W. (2023). Variation in seed protein, selected minerals, phytic acid, and potential mineral bioavailability of cowpea [Vigna unguiculata (L.) Walp] mutants and accessions. Crop Science, 1-15. https://doi.org/10.1002/csc2.21163
Núñez-Colín, C. A., & Escobedo-López, D. (2011). Uso correcto del análisis clúster en la caracterización de germoplasma vegetal. Agronomía mesoamericana, 22(2), 415-427.
Orozco-Ramírez, Q., Odenthal, J. & Astier, M. (2017). Diversidad de maíces en Pátzcuaro, Michoacán, México, y su relación con factores ambientales y sociales. Agrociencia, 51, 867-884.
Pang, J., Bansal, R., Zhao, H., Bohuon, E., Lambers, H., et al. (2018). The carboxylate‐releasing phosphorus‐mobilizing strategy can be proxied by foliar manganese concentration in a large set of chickpea germplasm under low phosphorus supply. New Phytologist, 219(2), 518-529. https://doi.org/10.1111/nph.15200
Perera, I., Fukushima, A., Arai, M., Yamada, K., Nagasaka, S., Seneweera, S., & Hirotsu, N. (2019). Identification of low phytic acid and high Zn bioavailable rice (Oryza sativa L.) from 69 accessions of the world rice core collection. Journal of Cereal Science, 85, 206-213. https://doi.org/10.1016/j.jcs.2018.12.010
Perera, I., Seneweera, S., & Hirotsu, N. (2018). Manipulating the phytic acid content of rice grain toward improving micronutrient bioavailability. Rice, 11, 1-13.
Raboy, V., Cichy, K., Peterson, K., Reichman, S., Sompong, U., et al. (2014). Barley (Hordeum vulgare L.) low phytic acid 1-1: an endosperm-specific, filial determinant of seed total phosphorus. Journal of Heredity, 105, 656-665. https://doi.org/10.1093/jhered/esu044
Raboy, V. (2020). Low phytic acid crops: observations based on four decades of research. Plants-Basel, 53, 27-36. https://doi.org/10.3390/plants9020140
Romesburg, H. C. (1984). Cluster Analysis for Researchers. Lifetime Learning Publications Belmont, California. 277 p.
SAS. (2011). SAS User's Guide: Statictics. SAS. Institute, Inc. Cary, North Carolina, USA.
Schneider, K. D., Thiessen Martens, J. R., Zvomuya, F., Reid, D. K., Fraser, T. D., Lynch, D. H., ... & Wilson, H. F. (2019). Options for improved phosphorus cycling and use in agriculture at the field and regional scales. Journal of Environmental Quality, 48(5), 1247-1264. https://doi.org/10.2134/jeq2019.02.0070
Stanton, C., Sanders, D., Krämer, U., & Podar, D. (2022). Zinc in plants: Integrating homeostasis and biofortification. Molecular Plant, 15(1), 65-85. https://doi.org/10.1016/j.molp.2021.12.008
Vaintraub, I. A., & Lapteva, N. A. (1988). Colorimetric determination of phytate in unpurified extracts of seeds and the products of their processing. Analytical Biochemistry, 175, 227-230. https://doi.org/10.1016/0003-2697(88)90382-X
Wang, F., Morrison, J. D., Rose, T., Kretzschmar, T., & Wissuwa, M. (2017). Can natural variation in grain P concentrations be exploited in rice breeding to lower fertilizer requirements? PLoS One, 12(6), 1-17. https://doi.org/10.1371/journal.pone.0179484
Wang, L., Cutforth, H., Lal, R., Chai, Q., Zhao, C., Gan, Y., & Siddique, K. H. (2018). ‘Decoupling’land productivity and greenhouse gas footprints: A review. Land Degradation & Development, 29(12), 4348-4361. https://doi.org/10.1002/ldr.3172
Wang, C., & Ning, P. (2019). Post-silking phosphorus recycling and carbon partitioning in maize under low to high phosphorus inputs and their effects on grain yield. Frontiers in plant science, 784, 1-13. https://doi.org/10.3389/fpls.2019.00784
Wang, X., He, Y., Gao, Q., Yang, D., & Liang, J. (2021). Approaches to evaluate nutrition of minerals in food. Food Science and Human Wellness, 10(2), 141-148. https://doi.org/10.1016/j.fshw.2021.02.002
Wu, D., Tanaka, R., Li, X., Ramstein, G. P., Cu, S., Hamilton, J. P., ... & Gore, M. A. (2021). High-resolution genome-wide association study pinpoints metal transporter and chelator genes involved in the genetic control of element levels in maize grain. G3, 11(4), jkab059. https://doi.org/10.1093/g3journal/jkab059
Zhao, H., Frank, T., Tan, Y., Zhou, C., Jabnoune, M., et al. (2016). Disruption of OsSULTR3; 3 reduces phytate and phosphorus concentrations and alters the metabolite profile in rice grains. New Phytologist, 211, 926-939. https://doi.org/10.1111/nph.13969
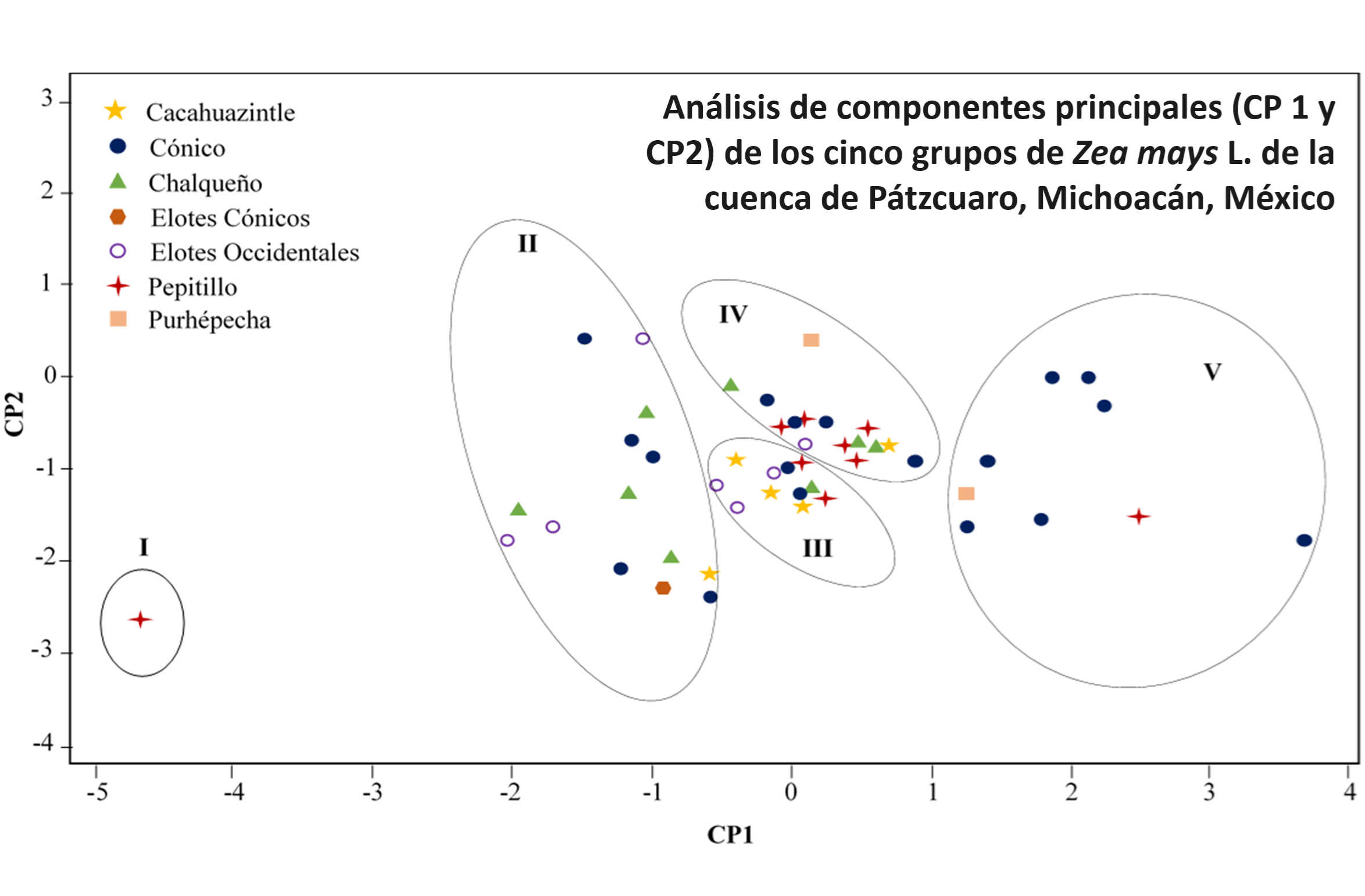
Downloads
Published
How to Cite
Issue
Section
License
Copyright (c) 2024 Scientia Agropecuaria

This work is licensed under a Creative Commons Attribution-NonCommercial 4.0 International License.
The authors who publish in this journal accept the following conditions:
a. The authors retain the copyright and assign to the magazine the right of the first publication, with the work registered with the Creative Commons attribution license, which allows third parties to use the published information whenever they mention the authorship of the work and the First publication in this journal.
b. Authors may make other independent and additional contractual arrangements for non-exclusive distribution of the version of the article published in this journal (eg, include it in an institutional repository or publish it in a book) as long as it clearly indicates that the work Was first published in this journal.
c. Authors are encouraged to publish their work on the Internet (for example, on institutional or personal pages) before and during the review and publication process, as it can lead to productive exchanges and a greater and faster dissemination of work Published (see The Effect of Open Access).