Biotechnological tools for genetic improvement of Trichoderma
DOI:
https://doi.org/10.17268/sci.agropecu.2024.016Palabras clave:
metabolites, genetic transformation, molecular tools, TrichodermaResumen
Trichoderma is a cosmopolitan fungus widely distributed around the world. The different uses of this beneficial fungus are varied in several industries, like agriculture, textile, and paper, including the pharmaceutical industry. The genus Trichoderma has other mechanisms of action, including the production of different enzymes and secreted secondary metabolites used in various industries. The genomes of different Trichoderma species have been sequenced to identify the mechanisms for producing several compounds. The advancement of multiple technologies has allowed the development of transformation tools for the genetic improvement of Trichoderma, thus increasing biomass, primary and secondary metabolites, and enzymes. Therefore, genetic modification aims to increase compound production in several Trichoderma strains. Characterization of Trichoderma through gene expression analysis is essential for biotechnology applications. It helps counteract one of the most challenging problems for agriculture today, including climate change and the appearance of pathogens that attack crops with high commercial and food demand. In conclusion, this review analyzes various strategies to improve Trichoderma strains genetically and their multiple applications in the agricultural, textile, paper, and pharmaceutical industries. As a recommendation for future studies with potential impact, the optimization of specific genetic modifications in Trichoderma strains is recommended to improve their adaptability and effectiveness in combating emerging challenges in agriculture, especially those linked to climate change. Investigating possible synergies between genetically modified Trichoderma strains and environmentally sustainable agricultural practices could contribute to developing solutions for crop protection and yield improvement.
Citas
Abd, A. A., Saleh, S. A. A., Eid, B. M., Ibrahim, N. A., & Mostafa, F. A. (2018). Thermodynamics characterization and potential textile applications of Trichoderma longibrachiatum KT693225 xylanase. Biocatal Agric Biotechnol, 14, 129-137. https://doi.org/10.1016/J.BCAB.2018.02.011
Abdul-Halim, A. M. A. A., Shivanand, P., Krishnamoorthy, S., & Taha, H. (2023). A review on the biological properties of Trichoderma spp. as a prospective biocontrol agent and biofertilizer. J Appl Biol Biotechnol, 11(5), 34-46 https://doi.org/10.7324/JABB.2023.11504
Arai, T., Wada, M., Nishiguchi, H., Takimura, Y., & Ishii, J. (2023). Inducer-free recombinant protein production in Trichoderma reesei: secretory production of endogenous enzymes and heterologous nanobodies using glucose as the sole carbon source. Microb Cell Fact, 22, 103. https://doi.org/10.1186/s12934-023-02109-y
Arazoe, T., Miyoshi, K., Yamato, T., Ogawa, T., Ohsato, S., Arie, T., & Kuwata, S. (2015). Tailor-made CRISPR/Cas system for highly efficient targeted gene replacement in the rice blast fungus. Biotechnol Bioeng, 112(12), 2543-2549. https://doi.org/10.1002/bit.25662
Atanasova, L., Knox, B. P., Kubicek, C. P., Druzhinina, I. S., & Baker, S. E. (2013). The polyketide synthase gene pks4 of Trichoderma reesei provides pigmentation and stress resistance. Eukaryot Cell, 12(11). https://doi.org/10.1128/EC.00103-13
Avilés, D., Espinoza, F., Villao, L., Alvarez, J., Sosa, D., Santos-Ordóñez, E., & Galarza, L. (2023). Application of microencapsulated Trichoderma spp. against Moniliophthora roreri during the vegetative development of cocoa. Scientia Agropecuaria, 14(4), 539-547. https://doi.org/10.17268/sci.agropecu.2023.045
Bae, Y. S., & Knudsen, G. R. (2000). Cotransformation of Trichoderma harzianum with β-glucuronidase and green fluorescent protein genes provides a useful tool for monitoring fungal growth and activity in natural soils. Appl Environ Microbiol, 66(2). https://doi.org/10.1128/AEM.66.2.810-815.2000
Cai, F., Kubicek, C. P., & Druzhinina, I. S. (2021). Genetic transformation of Trichoderma spp. In: Basu, C. (eds) Biofuels and Biodiesel. Methods in Molecular Biology, vol 2290. Humana, New York, NY. https://doi.org/10.1007/978-1-0716-1323-8_12
Çakmakçı, R., Salık, M. A., & Çakmakçı, S. (2023). Assessment and principles of environmentally sustainable food and agriculture systems. Agriculture, 13(5), 1073. https://doi.org/10.3390/agriculture13051073
Cardoza, R. E., Vizcaino, J. A., Hermosa, M. R., Monte, E., & Gutiérrez, S. (2006). A comparison of the phenotypic and genetic stability of recombinant Trichoderma spp. generated by protoplast - and Agrobacterium - mediated transformation. Journal of Microbiology, 44(4), 383-395.
Carvalho, E. A., dos Santos Góes, L. M., Uetanabaro, A. P. T., da Silva, E. G. P., Rodrigues, L. B., Pirovani, C. P., & da Costa, A. M. (2017). Thermoresistant xylanases from Trichoderma stromaticum: Application in bread making and manufacturing xylo-oligosaccharides. Food Chem, 221, 1499-1506. https://doi.org/10.1016/j.foodchem.2016.10.144
Chóez-Guaranda, I., Espinoza-Lozano, F., Reyes-Araujo, D., Romero, C., Manzano, P., Galarza, L., & Sosa, D. (2023). Chemical characterization of Trichoderma spp. extracts with antifungal activity against cocoa pathogens. Molecules, 28(7), 3208. https://doi.org/10.3390/molecules28073208
Chung, D., Kwon, Y. M., Lim, J. Y., Bae, S. S., Choi, G., & Lee, D. S. (2022). Characterization of chitinolytic and antifungal activities in marine-derived Trichoderma bissettii strains. Mycobiology, 50(4), 244-253. https://doi.org/10.1080/12298093.2022.2105509
Cortes, M. V. de C. B., Barbosa, E. T., Oliveira, M. I. de S., Maciel, L. H. R., Lobo Junior, M., et al. (2023). Trichoderma harzianum marker-free strain construction based on efficient CRISPR/Cas9 recyclable system: A helpful tool for the study of biological control agents. Biological Control, 184, 105281 https://doi.org/10.1016/j.biocontrol.2023.105281
De Paula, R. G., Antoniêto, A. C. C., Ribeiro, L. F. C., Carraro, C. B., Nogueira, K. M. V., et al. (2018). New genomic approaches to enhance biomass degradation by the industrial fungus Trichoderma reesei. Int J Genomics, 1974151. https://doi.org/10.1155/2018/1974151
Donzelli, B. G. G., Lorito, M., Scala, F., & Harman, G. E. (2001). Cloning, sequence and structure of a gene encoding an antifungal glucan 1,3-β-glucosidase from Trichoderma atroviride (T. harzianum). Gene, 277, 199-208. https://doi.org/10.1016/S0378-1119(01)00681-3
El-Saadony, M. T., Saad, A. M., Soliman, S. M., Salem, H. M., Ahmed, A. I., et al. (2022). Plant growth-promoting microorganisms as biocontrol agents of plant diseases: Mechanisms, challenges and future perspectives. Front Plant Sci, 13, 923880. https://doi.org/10.3389/fpls.2022.923880
Fazeli-Nasab, B., Shahraki-Mojahed, L., Piri, R., & Sobhanizadeh, A. (2022). Trichoderma: Improving growth and tolerance to biotic and abiotic stresses in plants, in: Trends of Applied Microbiology for Sustainable Economy. 525-564 https://doi.org/10.1016/B978-0-323-91595-3.00004-5
Ferreira Filho, J. A., Horta, M. A. C., dos Santos, C. A., Almeida, D. A., Murad, N. F., et al. (2020). Integrative genomic analysis of the bioprospection of regulators and accessory enzymes associated with cellulose degradation in a filamentous fungus (Trichoderma harzianum). BMC Genomics, 21, 757. https://doi.org/10.1186/s12864-020-07158-w
Fitz, E., Wanka, F., & Seiboth, B. (2018). The promoter toolbox for recombinant gene expression in Trichoderma reesei. Front Bioeng Biotechnol, 6, 135. https://doi.org/10.3389/FBIOE.2018.00135/BIBTEX
Galarza, L., Akagi, Y., Takao, K., Kim, C. S., Maekawa, N., et al. (2015). Characterization of Trichoderma species isolated in Ecuador and their antagonistic activities against phytopathogenic fungi from Ecuador and Japan. Journal of General Plant Pathology, 81, 201-210. https://doi.org/10.1007/S10327-015-0587-X/FIGURES/3
Gladek, E., Gerald, R., Muños Sabag, O., Kennedy, E., Fraser, M., & Hirsh, P. (2017). The Global Food System: An Analysis. Report commissioned by WWF Netherlands. Metabolic. https://www.metabolic.nl/publication/global-food-system-an-analysis/
Goldman, G. H., van Montagu, M., & Herrera-Estrella, A. (1990). Transformation of Trichoderma harzianum by high-voltage electric pulse. Curr Genet, 17, 169-174. https://doi.org/10.1007/BF00312863
Green, H., & Jensen, D. F. (1995). A tool for monitoring Trichoderma harzianum: II. The use of a GUS transformant for ecological studies in the rhizosphere. Phytopathology, 85, 1436-1440. https://doi.org/10.1094/Phyto-85-1436
Guo, Q., Shi, L., Wang, X., Li, D., Yin, Z., Zhang, J., Ding, G., & Chen, L. (2023). Structures and biological activities of secondary metabolites from the Trichoderma genus (Covering 2018-2022). J Agric Food Chem, 71(37), 13612-13632. https://doi.org/10.1021/acs.jafc.3c04540
Guzmán-Guzmán, P., Kumar, A., de los Santos-Villalobos, S., Parra-Cota, F. I., Orozco-Mosqueda, M. del C., et al. (2023). Trichoderma species: Our best fungal allies in the biocontrol of plant diseases - A Review. Plants, 12(3), 432 https://doi.org/10.3390/plants12030432
Hao, Z., & Su, X. (2019). Fast gene disruption in Trichoderma reesei using in vitro assembled Cas9/gRNA complex. BMC Biotechnol, 19, 2. https://doi.org/10.1186/s12896-018-0498-y
Harman, G. E., Howell, C. R., Viterbo, A., Chet, I., & Lorito, M. (2004). Trichoderma species - opportunistic, avirulent plant symbionts. Nat Rev Microbiol, 2, 43-56. https://doi.org/10.1038/NRMICRO797
Howell, C. R. (2003). Mechanisms employed by Trichoderma species in the biological control of plant diseases: The history and evolution of current concepts. Plant Disease, 87(1), 4-10. https://doi.org/10.1094/pdis.2003.87.1.4
Howell, C. R., Stipanovic, R. D., & Lumsden, R. D. (2008). Antibiotic production by strains of Gliocladium virens and its relation to the biocontrol of cotton seedling diseases. Biocontrol Science and Technology, 3(4), 435-441. https://doi.org/10.1080/09583159309355298
Iyyappan, J., Pravin, R., Al-Ghanim, K. A., Govindarajan, M., Nicoletti, M., & Baskar, G. (2023). Dual strategy for bioconversion of elephant grass biomass into fermentable sugars using Trichoderma reesei towards bioethanol production. Bioresour Technol, 374, 128804. https://doi.org/10.1016/j.biortech.2023.128804
Katayama, T., Tanaka, Y., Okabe, T., Nakamura, H., Fujii, W., Kitamoto, K., & Maruyama, J. (2016). Development of a genome editing technique using the CRISPR/Cas9 system in the industrial filamentous fungus Aspergillus oryzae. Biotechnol Lett, 38, 637-642. https://doi.org/10.1007/s10529-015-2015-x
Khan, R. A. A., Najeeb, S., Hussain, S., Xie, B., & Li, Y. (2020). Bioactive secondary metabolites from Trichoderma spp. Against phytopathogenic fungi. Microorganisms, 8(6), 817. https://doi.org/10.3390/microorganisms8060817
Kubicek, C. P., Steindorff, A. S., Chenthamara, K., Manganiello, G., Henrissat, B., Zhang, J., Cai, F., Kopchinskiy, A. G., Kubicek, E. M., Kuo, A., Baroncelli, R., Sarrocco, S., Noronha, E. F., Vannacci, G., Shen, Q., Grigoriev, I. V., & Druzhinina, I. S. (2019). Evolution and comparative genomics of the most common Trichoderma species. BMC Genomics, 20, 485 https://doi.org/10.1186/s12864-019-5680-7
Kück, U., & Hoff, B. (2010). New tools for the genetic manipulation of filamentous fungi. Appl Microbiol Biotechnol, 86, 51-62. https://doi.org/10.1007/s00253-009-2416-7
Li, D., Tang, Y., Lin, J., & Cai, W. (2017). Methods for genetic transformation of filamentous fungi. Microb Cell Fact, 16, 1-13. https://doi.org/10.1186/s12934-017-0785-7
Liu, D., Garrigues, S., & de Vries, R. P. (2023). Heterologous protein production in filamentous fungi. Appl Microbiol Biotechnol, 107, 5019-5033. https://doi.org/10.1007/s00253-023-12660-8
Liu, R., Chen, L., Jiang, Y., Zhou, Z., & Zou, G. (2015). Efficient genome editing in filamentous fungus Trichoderma reesei using the CRISPR/Cas9 system. Cell Discov, 1, 15007. https://doi.org/10.1038/celldisc.2015.7
Lorito, M., Hayes, C. K., di Pietro, A., & Harman, G. E. (1993). Biolistic transformation of Trichoderma harzianum and Gliocladium virens using plasmid and genomic DNA. Curr Genet, 24, 349-356. https://doi.org/10.1007/BF00336788
Magaña-Ortíz, D., Coconi-Linares, N., Ortiz-Vazquez, E., Fernández, F., Loske, A. M., & Gómez-Lim, M. A. (2013). A novel and highly efficient method for genetic transformation of fungi employing shock waves. Fungal Genetics and Biology, 56, 9-16. https://doi.org/10.1016/j.fgb.2013.03.008
Martín, J. F. (2015). Fungal transformation: From protoplasts to targeted recombination systems. In: van den Berg, M., Maruthachalam, K. (eds) Genetic Transformation Systems in Fungi, Volume 1. Fungal Biology. Springer, Cham. https://doi.org/10.1007/978-3-319-10142-2_1
Martinez, D., Berka, R. M., Henrissat, B., Saloheimo, M., Arvas, M., et al. (2008). Genome sequencing and analysis of the biomass-degrading fungus Trichoderma reesei (syn. Hypocrea jecorina). Nat Biotechnol, 26, 553-560. https://doi.org/10.1038/nbt1403
Maruyama, C. R., Bilesky-José, N., de Lima, R., & Fraceto, L. F. (2020). Encapsulation of Trichoderma harzianum preserves enzymatic activity and enhances the potential for biological control. Front Bioeng Biotechnol, 8, 225. https://doi.org/10.3389/fbioe.2020.00225
Mukherjee, P. K., Buensanteai, N., Moran-Diez, M. E., Druzhinina, I. S., & Kenerley, C. M. (2012a). Functional analysis of non-ribosomal peptide synthetases (NRPSs) in Trichoderma virens reveals a polyketide synthase (PKS)/NRPS hybrid enzyme involved in the induced systemic resistance response in maize. Microbiology, 158, 155-165. https://doi.org/10.1099/mic.0.052159-0
Mukherjee, P. K., Horwitz, B. A., Herrera-Estrella, A., Schmoll, M., & Kenerley, C. M. (2013). Trichoderma research in the Genome Era. Annual review of phytopathology, 51, 105-129. https://doi.org/10.1146/ANNUREV-PHYTO-082712-102353
Mukherjee, P. K., Horwitz, B. A., Kenerley, C. M. (2012b). Secondary metabolism in Trichoderma - A genomic perspective. Microbiology, 158, 35-45. https://doi.org/10.1099/mic.0.053629-0
Nogueira-López, G., Padilla-Arizmendi, F., Inwood, S., Lyne, S., Steyaert, J. M., Nieto-Jacobo, M. F., Stewart, A., & Mendoza-Mendoza, A. (2019). TrichoGate: An improved vector system for a large scale of functional analysis of Trichoderma genes. Front Microbiol, 10, 1-18. https://doi.org/10.3389/fmicb.2019.02794
Paloheimo, M., Haarmann, T., Mäkinen, S., & Vehmaanperä, J. (2016). Production of industrial enzymes in Trichoderma reesei. In: Schmoll, M., Dattenböck, C. (eds) Gene Expression Systems in Fungi: Advancements and Applications. Fungal Biology. Springer, Cham. https://doi.org/10.1007/978-3-319-27951-0_2
Pohl, C., Kiel, J. A. K. W., Driessen, A. J. M., Bovenberg, R. A. L., & Nygård, Y. (2016). CRISPR/Cas9 based genome editing of Penicillium chrysogenum. ACS Synth Biol, 5, 7, 754-764. https://doi.org/10.1021/acssynbio.6b00082
Qi, Q., Fan, C., Wu, H., Sun, L., & Cao, C. (2023). Preparation of Trichoderma asperellum microcapsules and biocontrol of cucumber powdery mildew. Microbiol Spectr, 11(3), e05084-22. https://doi.org/10.1128/spectrum.05084-22
Rantasalo, A., Vitikainen, M., Paasikallio, T., Jäntti, J., Landowski, C. P., & Mojzita, D. (2019). Novel genetic tools that enable highly pure protein production in Trichoderma reesei. Sci Rep, 9, 5032. https://doi.org/10.1038/s41598-019-41573-8
Rosmana, A., Taufik, M., Asman, A., Jayanti, N. J., & Hakkar, A. A. (2019). Dynamic of vascular streak dieback disease incidence on susceptible cacao treated with composted plant residues and Trichoderma asperellum in field. Agronomy, 9(10), 650. https://doi.org/10.3390/agronomy9100650
Rubio, M. B., Pardal, A. J., Cardoza, R. E., Gutiérrez, S., Monte, E., & Hermosa, R. (2017). Involvement of the transcriptional coactivator ThMBF1 in the biocontrol activity of Trichoderma harzianum. Front Microbiol, 8. https://doi.org/10.3389/fmicb.2017.02273
Sandle, T. (2014). Trichoderma. Encyclopedia of Food Microbiology: Second Edition, 644-646. https://doi.org/10.1016/B978-0-12-384730-0.00337-2
Saravanakumar, K., Rathnayake, A. U., Sathiyaseelan, A., Selvakumar, V., Vijaya Anand, M. A., et al. (2020). Trichoderma-functional metabolomics to genetic engineering. New and Future Developments in Microbial Biotechnology and Bioengineering, 209-220. https://doi.org/10.1016/B978-0-12-819453-9.00010-6
Schalamun, M., & Schmoll, M. (2022). Trichoderma - genomes and genomics as treasure troves for research towards biology, biotechnology and agriculture. Frontiers in Fungal Biology, 3. https://doi.org/10.3389/ffunb.2022.1002161
Schmoll, M., & Schuster, A. (2010). Biology and biotechnology of Trichoderma. Appl Microbiol Biotechnol, 87, 787-799. https://doi.org/10.1007/s00253-010-2632-1
Schuster, M., Schweizer, G., Reissmann, S., & Kahmann, R. (2016). Genome editing in Ustilago maydis using the CRISPR-Cas system. Fungal Genetics and Biology, 89, 3-9. https://doi.org/10.1016/j.fgb.2015.09.001
Shi, T. Q., Liu, G. N., Ji, R. Y., Shi, K., Song, P., Ren, L. J., Huang, H., & Ji, X. J. (2017). CRISPR/Cas9-based genome editing of the filamentous fungi: the state of the art. Appl Microbiol Biotechnol, 101, 7435-7443. https://doi.org/10.1007/s00253-017-8497-9
Simamora, M., Basyuni, M., & Lisnawita, (2021). Potency of secondary metabolites of Trichoderma asperellum and Pseudomonas fluorescens in the growth of cocoa plants affected by vascular streak dieback. Biodiversitas, 22(5), 2542-2547. https://doi.org/10.13057/biodiv/d220511
Steen, E. J., Kang, Y., Bokinsky, G., Hu, Z., Schirmer, A., McClure, A., del Cardayre, S. B., & Keasling, J. D. (2010). Microbial production of fatty-acid-derived fuels and chemicals from plant biomass. Nature, 463, 559-562. https://doi.org/10.1038/nature08721
Stoppacher, N., Kluger, B., Zeilinger, S., Krska, R., & Schuhmacher, R. (2010). Identification and profiling of volatile metabolites of the biocontrol fungus Trichoderma atroviride by HS-SPME-GC-MS. J Microbiol Methods, 81(2), 187-193. https://doi.org/10.1016/j.mimet.2010.03.011
Stracquadanio, C., Quiles, J. M., Meca, G., & Cacciola, S. O. (2020). Antifungal activity of bioactive metabolites produced by Trichoderma asperellum and Trichoderma atroviride in liquid medium. Journal of Fungi, 6(4), 263. https://doi.org/10.3390/jof6040263
Suarez, B., Rey, M., Castillo, P., Monte, E., & Llobell, A. (2004). Isolation and characterization of PRA1, a trypsin-like protease from the biocontrol agent Trichoderma harzianum CECT 2413 displaying nematicidal activity. Appl Microbiol Biotechnol, 65, 46-55. https://doi.org/10.1007/S00253-004-1610-X
Szabó, M., Urbán, P., Virányi, F., Kredics, L., & Fekete, C. (2013). Comparative gene expression profiles of Trichoderma harzianum proteases during in vitro nematode egg-parasitism. Biological Control, 67, 337-343. https://doi.org/10.1016/J.BIOCONTROL.2013.09.002
Tyśkiewicz, R., Nowak, A., Ozimek, E., & Jaroszuk-ściseł, J. (2022). Trichoderma: The current status of its application in agriculture for the biocontrol of fungal phytopathogens and stimulation of plant growth. Int J Mol Sci, 23(4), 2329. https://doi.org/10.3390/ijms23042329
Valenzuela-Cobos, J. D., Guevara-Viejó, F., Vicente-Galindo, P., & Galindo-Villardón, P. (2023). Eco-Friendly Biocontrol of Moniliasis in Ecuadorian Cocoa Using Biplot Techniques. Sustainability, 15(5), 4223. https://doi.org/10.3390/su15054223
Vieira, A. A., Vianna, G. R., Carrijo, J., Aragão, F. J. L., & Vieira, P. M. (2021). Generation of Trichoderma harzianum with pyr4 auxotrophic marker by using the CRISPR/Cas9 system. Sci Rep, 11, 1085. https://doi.org/10.1038/s41598-020-80186-4
Wang, Q., Chen, L., Fang, C., Wang, H., Shi, Y., & Zhao, Y. (2019). The overexpression of one single cbh gene making Trichoderma asperellum T-1 a better cellulase producer. Ann Microbiol, 69, 673-683. https://doi.org/10.1007/s13213-019-01458-7
Wang, W., Shi, X. Y., & Wei, D. Z. (2014). Light-mediated control of gene expression in filamentous fungus Trichoderma reesei. J Microbiol Methods, 103, 37-39. https://doi.org/10.1016/j.mimet.2014.05.017
Wang, Y., Chen, H., Ma, L., Gong, M., Wu, Y., Bao, D., & Zou, G. (2022). Use of CRISPR-Cas tools to engineer Trichoderma species. Microb Biotechnol, 15, 2521-2532. https://doi.org/10.1111/1751-7915.14126
Wiest, A., Grzegorski, D., Xu, B. W., Goulard, C., Rebuffat, S., Ebbole, D. J., Bodo, B., & Kenerley, C. (2002). Identification of peptaibols from Trichoderma virens and cloning of a peptaibol synthetase. Journal of Biological Chemistry, 277(23), 20862-20868. https://doi.org/10.1074/jbc.M201654200
Wilson, R. A., & Talbot, N. J. (2009). Fungal physiology - A future perspective. Microbiology, 155(12), 3810-3815. https://doi.org/10.1099/mic.0.035436-0
Wu, C., Chen, Y., Huang, X., Sun, S., Luo, J., Lu, Z., Wang, W., & Ma, Y. (2019). An efficient shortened genetic transformation strategy for filamentous fungus Trichoderma reesei. Journal of General and Applied Microbiology, 65(6), 301-307. https://doi.org/10.2323/jgam.2019.02.001
Wu, C., Chen, Y., Qiu, Y., Niu, X., Zhu, N., Chen, J., Yao, H., Wang, W., & Ma, Y. (2020). A simple approach to mediate genome editing in the filamentous fungus Trichoderma reesei by CRISPR/Cas9-coupled in vivo gRNA transcription. Biotechnol Lett, 42, 1203-1210. https://doi.org/10.1007/s10529-020-02887-0
Xu, Q., Himmel, M. E., & Singh, A. (2015). Production of ethanol from engineered Trichoderma reesei. Direct microbial conversion of biomass to advanced biofuels, 197-208. https://doi.org/10.1016/B978-0-444-59592-8.00011-7
Yao, L., Tan, C., Song, J., Yang, Q., Yu, L., & Li, X. (2016). Isolation and expression of two polyketide synthase genes from Trichoderma harzianum 88 during mycoparasitism. Brazilian Journal of Microbiology, 47(2), 468-479. https://doi.org/10.1016/j.bjm.2016.01.004
Yao, X., Guo, H., Zhang, K., Zhao, M., Ruan, J., & Chen, J. (2023). Trichoderma and its role in biological control of plant fungal and nematode disease. Front Microbiol, 14. https://doi.org/10.3389/fmicb.2023.1160551
Yazawa, H., Kumagai, H., & Uemura, H. (2013). Secretory production of ricinoleic acid in fission yeast Schizosaccharomyces pombe. Appl Microbiol Biotechnol, 97, 8663-8671. https://doi.org/10.1007/s00253-013-5060-1
Zeilinger, S. (2004). Gene disruption in Trichoderma atroviride via Agrobacterium-mediated transformation. Curr Genet, 45. https://doi.org/10.1007/s00294-003-0454-8
Zeilinger, S., Gruber, S., Bansal, R., & Mukherjee, P. K. (2016). Secondary metabolism in Trichoderma - Chemistry meets genomics. Fungal Biol Rev, 30(2), 74-90. https://doi.org/10.1016/j.fbr.2016.05.001
Zhang, C., Meng, X., Wei, X., & Lu, L. (2016). Highly efficient CRISPR mutagenesis by microhomology-mediated end joining in Aspergillus fumigatus. Fungal Genetics and Biology, 86. https://doi.org/10.1016/j.fgb.2015.12.007
Zhang, J. L., Tang, W. L., Huang, Q. R., Li, Y. Z., Wei, M. L., et al. (2021). Trichoderma: A treasure house of structurally diverse secondary metabolites with medicinal importance. Front Microbiol, 12. https://doi.org/10.3389/fmicb.2021.723828
Zhang, L., Zhao, X., Zhang, G., Zhang, J., Wang, X., Zhang, S., Wang, W., & Wei, D. (2016). Light-inducible genetic engineering and control of non-homologous end-joining in industrial eukaryotic microorganisms: LML 3.0 and OFN 1.0. Sci Rep, 6, 20761. https://doi.org/10.1038/srep20761
Zhang, Y., Adams, I. P., & Ratledge, C. (2007) Malic enzyme: the controlling activity for lipid production? Overexpression of malic enzyme in Mucor circinelloides leads to a 2.5-fold increase in lipid accumulation. Microbiology, 153, 2013-2025. https://doi.org/10.1099/MIC.0.2006/002683-0
Zin, N., & Badaluddin, N. (2020). Biological functions of Trichoderma spp. for agriculture applications. Annals of Agricultural Sciences, 65(2), 168-178. https://doi.org/10.1016/j.aoas.2020.09.003
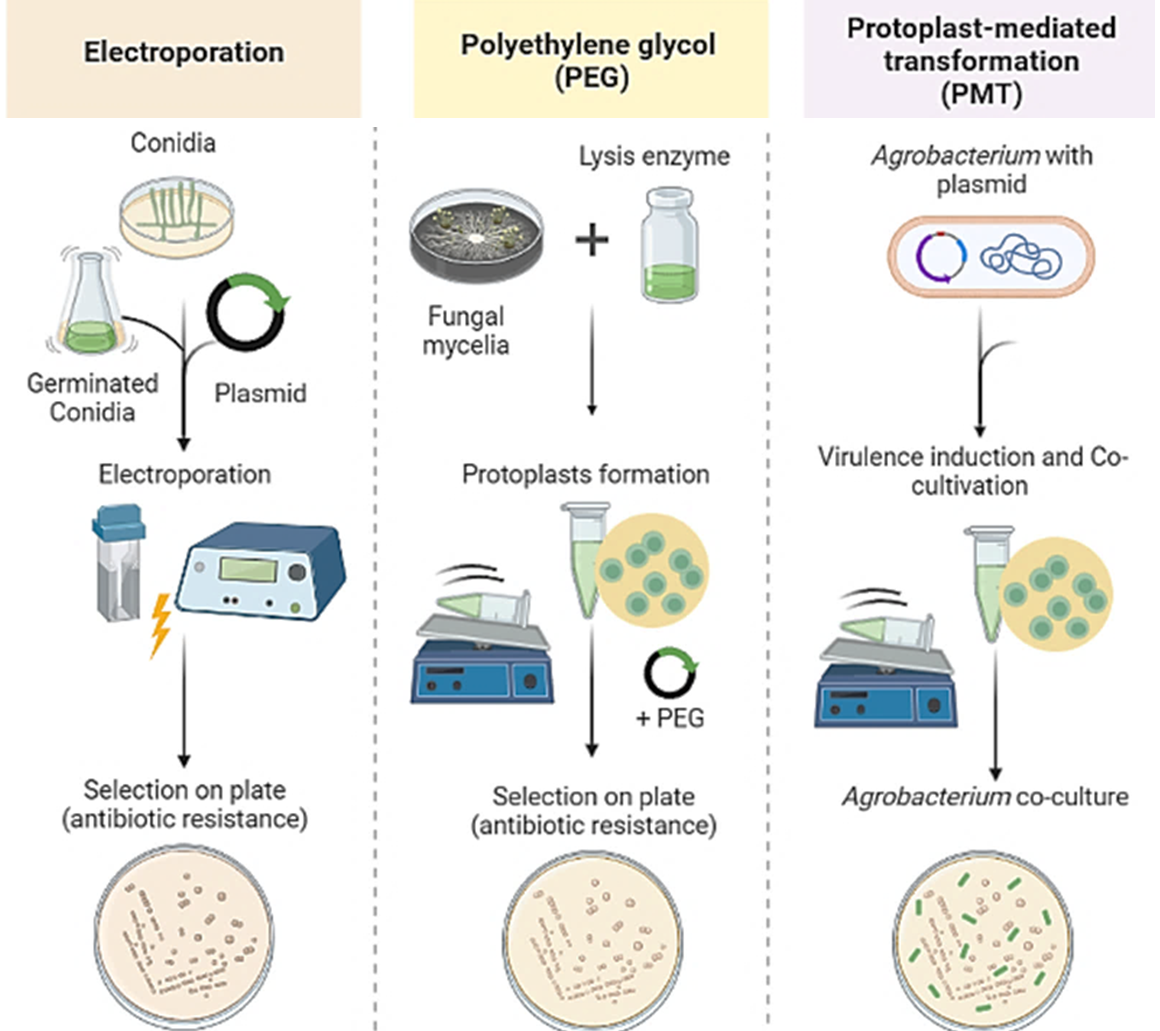
Descargas
Publicado
Cómo citar
Número
Sección
Licencia
Derechos de autor 2024 Scientia Agropecuaria

Esta obra está bajo una licencia internacional Creative Commons Atribución-NoComercial 4.0.
Los autores que publican en esta revista aceptan los siguientes términos:
a. Los autores conservan los derechos de autor y conceden a la revista el derecho publicación, simultáneamente licenciada bajo una licencia de Creative Commons que permite a otros compartir el trabajo, pero citando la publicación inicial en esta revista.
b. Los autores pueden celebrar acuerdos contractuales adicionales separados para la distribución no exclusiva de la versión publicada de la obra de la revista (por ejemplo, publicarla en un repositorio institucional o publicarla en un libro), pero citando la publicación inicial en esta revista.
c. Se permite y anima a los autores a publicar su trabajo en línea (por ejemplo, en repositorios institucionales o en su sitio web) antes y durante el proceso de presentación, ya que puede conducir a intercambios productivos, así como una mayor citación del trabajo publicado (ver efecto del acceso abierto).